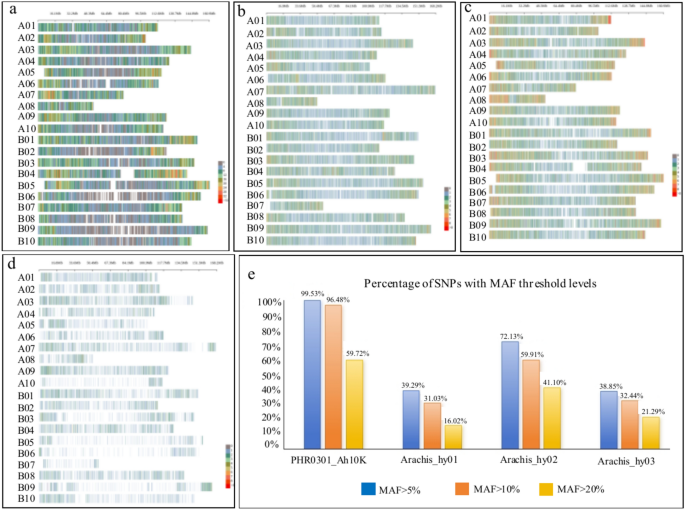
Dhillon, S. S. et al. Reassociation kinetics and cytophotometric characterization of peanut (Arachis hypogaea L.) DNA. Plant Physiol. 65, 1121–1127 (1980).
Win, M. M. et al. Phenolic compounds and antioxidant exercise of peanut’s pores and skin, hull, uncooked kernel and roasted kernel flour. Pak. J. Bot. 43, 1635–1642 (2011).
Pasupuleti, J. et al. Groundnut enchancment: use of genetic and genomic instruments. Entrance. Plant Sci. 4, 785. https://doi.org/10.3389/fpls.2013.00023 (2013).
Radhakrishnan, R. et al. Analysis of luteolin from shells of Korean peanut cultivars for industrial utilization. Afr. J. Biotechnol. 12, 4477–4480 (2013).
Musa, Ö. M. et al. Some dietary traits of kernel and oil of peanut (Arachis hypogaea L.). J. Oleo Sci. 59, 1–5 (2010).
Smartt, J. et al. The genomes of Arachis hypogaea L. Cytogenetic research of putative genome donors. Euphytica 27, 665–675 (1978).
Seijo, G. et al. Genomic relationships between the cultivated peanut (Arachis hypogaea, L.) and its shut relations revealed by double GISH. Am. J. Bot. 94, 1963–1971 (2007).
Robledo, G. et al. Species relations amongst wild Arachis species with the A genome as revealed by FISH mapping of rDNA loci and heterochromatin detection. Theor. Appl. Genet. 118, 1295–1307 (2009).
Bertioli, D. J. et al. The genome sequences of Arachis duranensis and Arachis ipaensis, the diploid ancestors of cultivated peanut. Nat. Genet. 48, 438–446 (2016).
Moretzsohn, M. C. et al. A research of the relationships of cultivated peanut (Arachis hypogaea) and its most carefully associated wild species utilizing intron sequences and microsatellite markers. Ann. Bot. 111, 113–126 (2012).
Nielen, S. et al. Matita, a brand new retroelement from peanut: characterization and evolutionary context within the mild of the Arachis A-B genome divergence. Mol. Genet. Genom. 287, 21–38 (2012).
Kim, Okay. S. et al. Improvement of SNP-based molecular markers by re-sequencing technique in peanut. Plant Breed. Biotechnol. 5, 325–333 (2017).
Chen, X. et al. Draft genome of the peanut A-genome progenitor (Arachis duranensis) offers insights into geocarpy, oil biosynthesis, and allergens. Proc. Natl. Acad. Sci. 113, 6785–6790 (2016).
Yang, H. et al. Utility of next-generation sequencing for fast marker growth in molecular plant breeding: a case research on anthracnose illness resistance in Lupinus angustifolius L.. BMC Genom. 13, 318 (2012).
Lee, J. et al. Genome-wide SNP identification and QTL mapping for black rot resistance in cabbage. BMC Plant Biol. 15, 32 (2015).
Kang, Y. J. et al. Resequencing of Capsicum annuum parental strains (YCM334 and Taean) for the genetic evaluation of bacterial wilt resistance. BMC Plant Biol. 16, 235 (2016).
Pandey, M. Okay. et al. Advances in Arachis genomics for peanut enchancment. Biotechnol. Adv. 30, 639–651 (2012).
Varshney, R. Okay. et al. Achievements and prospects of genomics-assisted breeding in three legume crops of the semi-arid tropics. Biotechnol. Adv. 31, 1120–1134 (2013).
Pandey, M. Okay. et al. Rising genomic instruments for legume breeding: present standing and future prospects. Entrance. Plant Sci. 7, 455 (2016).
Otyama, P. I. et al. Analysis of linkage disequilibrium, inhabitants construction, and genetic variety within the US peanut mini core assortment. BMC Genom. 20, 481 (2019).
Peng, Z. et al. Comparability of SNP calling pipelines and NGS platforms to foretell the genomic areas harboring candidate genes for nodulation in cultivated peanut. Entrance. Genet. 11, 222 (2020).
Zou, Okay. et al. Genetic variety and genome-wide affiliation research of seed facet ratio utilizing a high-density SNP array in peanut (Arachis hypogaea L.). Genes 12, 1. https://doi.org/10.3390/genes12010002 (2021).
McCouch, S. R. et al. Improvement of genome-wide SNP assays for rice. Breed. Sci. 60, 524–535 (2010).
Chen, H. et al. A excessive density SNP genotyping array for rice biology and molecular breeding. Mol. Plant. 7, 541–553 (2014).
Ganal, M. W. et al. A big maize (Zea mays L.) SNP genotyping array: growth and germplasm genotyping, and genetic mapping to match with the B73 reference genome. PLoS One 6, e28334 (2011).
Unterseer, S. et al. A strong instrument for genome evaluation in maize: growth and analysis of the excessive density 600 okay SNP genotyping array. BMC Genomics 15, 823 (2014).
Bachlava, E. et al. SNP discovery and growth of a high-density genotyping array for sunflower. PLoS One 7, e29814 (2012).
Music, Q. et al. Improvement and analysis of SoySNP50K, a high-density genotyping array for soybean. PLoS One 8, e54985 (2013).
Tinker, N. A. et al. A SNP genotyping array for hexaploid oat. Plant Genome 7, 3. https://doi.org/10.3835/plantgenome2014.03.0010 (2014).
Hulse-Kemp, A. et al. Improvement of a 63K SNP array for cotton and high-density mapping of intraspecific and interspecific populations of Gossypium spp. G3 (Bethesda) 5, 1187–1209 (2015).
Wang, S. et al. Characterization of polyploid wheat genomic variety utilizing a high-density 90,000 single nucleotide polymorphism array. Plant Biotechnol. J. 12, 787–796 (2014).
Winfield, M. O. et al. Excessive-density SNP genotyping array for hexaploid wheat and its secondary and tertiary gene pool. Plant Biotechnol. J. 14, 1195–1206 (2016).
Liu, Y. et al. GenoBaits Soy40K: a extremely fexible and lowcost SNP array for soybean research. Sci. China Life Sci. 65, 1898–1901 (2022).
Xu, Y. B. et al. Genotyping by goal sequencing (GBTS) and its purposes (in Chinese language). Sci. Agric. Sin. 53, 2983–3004 (2020).
Guo, Z. et al. Improvement of a number of SNP marker panels afordable to breeders by genotyping by goal sequencing (GBTS) in maize. Mol. Breed. 39, 3. https://doi.org/10.1007/s11032-019-0940-4 (2019).
Hussain, I. et al. Identifcation of heterotic teams and patterns primarily based on genotypic and phenotypic traits amongst rice accessions of various origins. Entrance. Genet. 13, 78. https://doi.org/10.3389/fgene.2022.811124 (2022).
Huang, S. et al. Epistatic interplay efect between chromosome 1BL (Yr29) and a novel locus on 2AL facilitating resistance to stripe rust in Chinese language wheat Changwu 357–9. Theor. Appl. Genet. 135, 2501–2513 (2022).
Wang, X. et al. Utilizing machine studying to enhance the accuracy of genomic prediction of replica traits in pigs. J. Anim. Sci. Biotechnol. 13, 1. https://doi.org/10.1186/s40104-022-00708-0 (2022).
Chen, X. et al. Sequencing of cultivated peanut, Arachis hypogaea, yields insights into genome evolution and oil enchancment. Mol. Plant. 12, 920–934 (2019).
Solar, Z. et al. Improvement and analysis of the utility of GenoBaits Peanut 40K for a peanut MAGIC inhabitants. Mol. Breed. 43, 10. https://doi.org/10.1007/s11032-023-01417-w (2023).
Chen, S. F. et al. fastp: an ultra-fast all-in-one FASTQ preprocessor. Bioinformatics 34, 145. https://doi.org/10.1093/bioinformatics/bty560 (2018).
Li, H. et al. Quick and correct brief learn alignment with Burrows-Wheeler remodel. Bioinformatics 25, 1754. https://doi.org/10.1093/bioinformatics/btp324 (2009).
Li, H. et al. Te Sequence Alignment/Map (SAM) Format and SAMtools. Bioinformatics 25(2078–2079), 45 (2009).
Picard instruments. https://broadinstitute.githu.bio/picard/ (2016).
Poplin, R. et al. A common SNP and small-indel variant caller utilizing deep neural networks. Nat. Biotechnol. 36, 983. https://doi.org/10.1038/nbt.4235 (2018).
Kumar, S. et al. MEGA X: molecular evolutionary genetics evaluation throughout computing platforms. Mol. Biol. Evol. 35, 1547–1549 (2018).
Nei, M. et al. Sampling variances of heterozygosity and genetic distance. Genetics 76, 379. https://doi.org/10.1093/genetics/76.2.379 (1974).
Botstein, et al. Building of a genetic linkage map in man utilizing restriction fragment size polymorphism. Am. J. Hum. Genet.32, 314–331 (1980)
Serrote, C. M. L. et al. Figuring out the polymorphism info content material of a molecular marker. Gene 726, 14415. https://doi.org/10.1016/j.gene.2019.144175 (2020).
Lu, Q. et al. A genomic variation map offers insights into peanut variety in China and associations with 28 agronomic traits. Nat. Genet. 56, 530–540 (2024).
Alexander, D. H. et al. Quick model-based estimation of ancestry in unrelated people. Genome Res. 19, 1655–1664 (2009).
Yang, et al. GCTA: a instrument for genome-wide complicated trait evaluation. Am. J. Hum. Genet. 88, 76–82 (2011).
Weir, B. et al. Genetic relatedness evaluation: trendy information and new challenges. Nat. Rev. Genet. 7, 771–780 (2006).
Kristen L. et al. Interpretation of DNA typing outcomes for Kinship evaluation. In Nationwide Institute of Requirements and Expertise USCIS Working Group on DNA Coverage. http://www.cstl.nist.gov/strbase/NISTpub.htm. (2011)
Zhang, C. et al. PopLDdecay: a quick and efficient instrument for linkage disequilibrium decay evaluation primarily based on variant name format information. Bioinformatics 35, 1786–1788 (2019).
Guo, et al. Improvement of high-resolution a number of SNP arrays for genetic analyses and molecular breeding by genotyping by goal sequencing and liquid chip. Plant Commun. 2, 61. https://doi.org/10.1016/j.xplc.2021.100230 (2001).
Liu, M. J. et al. Genetic variety of Shaanxi soybean landraces primarily based on agronomic traits and SSR markers. Afr. J. Biotechnol. 10, 4823–4837 (2011).
Afolayan, G. et al. Genetic variety evaluation of sorghum (Sorghum bicolor (L.) Moench) accessions utilizing single nucleotide polymorphism markers. Plant Genet. Resour. 17, 412–420 (2019).
Enyew, M. et al. Genetic variety and inhabitants construction of sorghum [Sorghum bicolor (L.) Moench] accessions as revealed by single nucleotide polymorphism markers. Entrance. Plant Sci. 2, 799482. https://doi.org/10.3389/fpls.2021.799482 (2022).
Yahaya, M. A. et al. Genetic variety and inhabitants construction of African Sorghum (Sorghum bicolor L. Moench) accessions assessed by single nucleotide polymorphisms markers. Genes 14, 1480. https://doi.org/10.3390/genes14071480 (2023).
Remington, D. L. et al. Construction of hyperlink age disequilibrium and phenotypic associations within the maize genome. Proc. Natl. Acad. Sci. 98, 11479–11484 (2001).
Flint-Garcia, S. A. et al. Construction of linkage disequilibrium in crops. Annu. Rev. Plant Biol. 54, 357–374 (2003).
Van Inghelandt, D. et al. Extent and genome-wide distribution of linkage disequilibrium in industrial maize germplasm. Theor. Appl. Genet. 123, 11–20 (2011).
Beckett, T. J. et al. Genetic relatedness of beforehand plant-variety protected industrial maize inbreds. PLoS One. 12, 12. https://doi.org/10.1371/journal.pone (2017).
Yan, J. et al. Genetic characterization and linkage disequilibrium estimation of a worldwide maize assortment utilizing SNP markers. PLoS One. 4, 52. https://doi.org/10.1371/journal.pone (2009).
Smith, A. V. et al. Sequence options in areas of weak and robust linkage disequilibrium. Genome Res. 15, 1519–1534 (2005).